Plasmas tend to be quite hot. This is because they consist of electrons and ions, which are strongly attracted to each other. To over come this attraction, the system must have sufficient kinetic energy, or more loosely, the particles need to be going ‘fast enough’. In my work, temperature is simply a measure of how fast, on average, the particles in a system are going. It’s a measure of the disordered, chaotic speeds in a system. An analogy is a cold, fast flowing river – all the particles are moving quickly in one direction, so the kinetic energy is large, but the temperature or thermal energy is still not very high. If this river hits a dam and slows down, most of that directed kinetic energy will go into heating up the water – the particles will move more randomly, and the temperature is now higher, but the water doesn’t flow because the speeds are all in random directions.
So how hot is a plasma? It’s possible to quickly calculate a temperature for a simple system of just one electron and one proton that are attracted to each other but kept apart by their large thermal energy. This tends to overestimate the temperature necessary for a plasma, but roughly 10,000 C is about when plasmas begin to form. At this temperature, the difference between degrees Kelvin and degrees Celsius is negligible – if you’re a fan of Fahrenheit, multiply by two and you won’t be far wrong.
This is quite hot, and sticking a mercury thermometer in and trying to measure off the scale isn’t really practical – the plasma would most likely loose all its energy to the thermometer, and even if it didn’t, the mercury would boil, evaporate and ionise and form a plasma that would leave you back where you started.
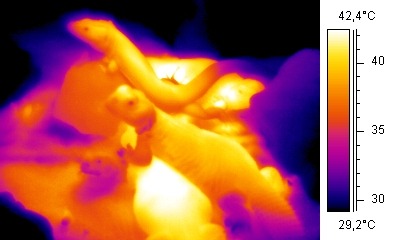
“Wiki lizards” by Arno / Coen Licensed under CC BY-SA 3.0
You could try one of those nifty infra-red cameras, beloved of spy films and Federal agents trying to spot grow rooms. These work by detecting black-body radiation, the concept that an object at a certain temperature emits light of specific frequencies (if you like, think ‘colour’ for frequency). Our plasma is so hot that it doesn’t glow in the infra-red, or even orange or white like a hot poker, but instead it glows with x-ray light, invisible to humans. Unfortunately, this isn’t a very precise diagnostic, as if different bits of our plasma are at different temperatures then we will just get a mish-mash of light at different frequencies.
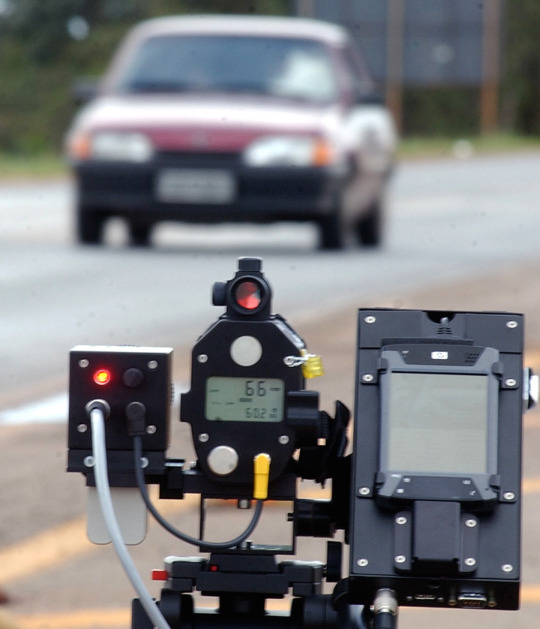
Credit: Fábio Pozzebom/ABr Licensed under CC BY 3.0
No, what we need is something more like a speed camera, specifically a Radar gun. These fire out radar waves that bounce off the speeding object and back to the source. The waves coming back are at a different frequency than the waves that were emitted – you’re probably familiar with this ‘Doppler shift’ from ambulances speeding by with their sirens on. When the ambulance is coming towards you the pitch is higher, and when moving away from you it sounds like a lower pitch. The same effect can happen with all waves, including light, and so we should be able to bounce waves off individual electrons and ions and see how fast they are going.
At this point, you might reasonably object that a car is somewhat bigger than an electron and the whole plan probably won’t work with a standard Radar gun. You’re quite right – what we really need is a very, very powerful light source, like a laser, that we can focus down to a tiny spot so that even though the chances of any of the light bouncing off an electron is quite small, we have such a high intensity to start with that the bounced (physicists say ‘scattered’) light is still detectable. Additionally, we are not bouncing light off one electron, but a blob of plasma containing billions upon billions of electrons, all of which bounce light back at us so that we can collect a measurable amount in our detectors.
What do we expect to see? If all the electrons are going in the same direction at the same speed, the light we collect will simply be at a slightly different frequency to the light we emitted. In reality, all the electrons are moving in different directions at different speeds – that’s what having a temperature means, random speeds in random directions. So we’ll collect light at a range of frequencies, and by looking at the range we have we can estimate the temperature of the plasma. This technique is called Thomson Scattering, and it’s widely used in plasma physics.
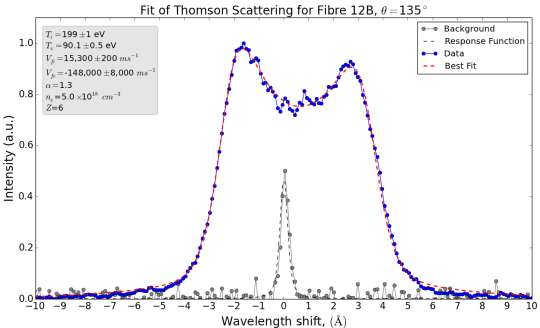
This plasma is around one million degrees Celsius. The laser we fired into the plasma has a well defined frequency – the light we collect has a broader range of frequencies. The analysis here is far from straightforwards – a simple model would just have a broader peak, and not two peaks of different heights.
In practice, when we examine the frequencies of the light we’ve collected, we might be quite surprised that it doesn’t follow the simple model above. This is because the plasma is made of charged particles, and the light is a tangle of electric and magnetic fields that push and pull the plasma around and are wiggled and wobbled in return. I’ll address this in my next blog post.